TRISO Particle FUEL
Uranium bearing spheres
the size of poppyseeds
A TRi-structural ISOtropic (TRISO) particle is a Uranium bearing sphere, the size of a poppyseed, coated with special ceramic layers designed like tiny pressure vessels. The layers contain fission products inside and ensure mechanical and chemical stability during irradiation and temperature changes. The fuel is extremely robust, with over 60 years of development and experience.
Why TRISO?
TRISO particle fuel is fundamentally different from conventional fuels which are centimeter sized pellets of Uranium Dioxide enclosed in 4-meter-long zirconium alloy tubes. The driving idea behind TRISO particle fuel is to give each millimeter sized piece of nuclear fuel its own containment and pressure vessel, significantly enhancing the fuel’s ability to contain fission products even up to very high temperatures and neutron bombardment where conventional fuel would melt or fail. The physical basis for miniaturizing the pressure vessel is twofold: fuel subdivision and pressure vessel enhancement.
Fuel subdivision eliminates risk of single point failure
Compared to normal fuel elements, a TRISO particle contains 5 million times less fissionable mass. Subdividing the nuclear fuel into discrete and self-containing packets eliminates the risk of single point failures in the pressure vessel or fuel cladding. Ordinarily, a single failure in the reactor’s main steel pressure vessel would lead to a nuclear accident. Even a single failure in a conventional fuel cladding results in leakage of fission products into the coolant and the vessel that can result in an unplanned outage or worse. With TRISO, single failures are unlikely, and when they occur, lead to a small radiation release into the fuel matrix where it is likely stopped. The subdivision of fuel into particles is even believed to provide blast resistance as the particles are small and robust enough to remain intact during explosive fractures like a direct missile hit.
Refractory ceramics for seamless, spherical pressure vessels
The second advantage to miniaturizing the pressure vessel is the ability to enhance pressure vessel performance through use of thin layered ceramics in a mass manufactured, seamless, spherical design. Fabrication of millions of particle pressure vessels can be highly standardized and controlled in a mass manufacturing environment with defect rates of less than 1 in 100,000. Crucially, the millimeter scale vessel allows the use of highly pure ceramics made through CVD techniques that maintain high strength and stability under high temperature and irradiation and have dual use as low reactivity fission product barriers. Normal reactor pressure vessels are made one at a time and have to be cylindrical, with various welding and joining techniques, multi cm wall thickness, and cannot be made from ceramics, instead using metals that melt. As the pressure vessel size is reduced, the more efficient spherical geometry can be adopted, and the required wall thickness drops dramatically to the point that a 35 μm SiC layer can indefinitely contain gas pressures in excess of 100 MPa. This compares to the main reactor steel pressure vessel which may go as high as 20MPa or the Zircalloy cladding which can have pressures up to 10s of MPa in limited conditions – temperatures far below what the ceramic pressure vessel tolerate. As the nuclear fuel fissions, it continues to accumulate fission product gases and increases the gas pressure that must be contained. If the pressure vessel can handle higher pressures, the fuel can be burned more extensively and safely, which means more efficient use of fuel.
The attractiveness of TRISO-matrix fuel is derived from its ceramics' unusually high thermal conductivity, high fission product retention, and superior corrosion resistance across operating, accident, and storage conditions. This translates into better economic potential and safety for current civilian reactors (higher temperatures, burnups, and power densities with higher safety margins) as well as upcoming micro reactors, gas cooled reactors, and molten salt cooled reactors. More extreme performance applications enabled by TRISO-matrix type concepts include nuclear thermal propulsion (NTP) for space, very high temperature reactors, and nuclear ramjets (i.e. nuclear powered aircraft).
TRISO Particle Layers
Meticulously Designed
The kernels are coated with a series of engineered ceramic layers using chemical vapor deposition. The thickness, porosity, grain size, and texture are strictly controlled to achieve the desired performance. SiC and graphite layers which offer excellent radiation and temperature tolerance, maintaining their performance characteristics across the intended temperature and irradiation conditions. The melting temperatures of SiC and graphite are about 2800 °C and 3800 °C, respectively.
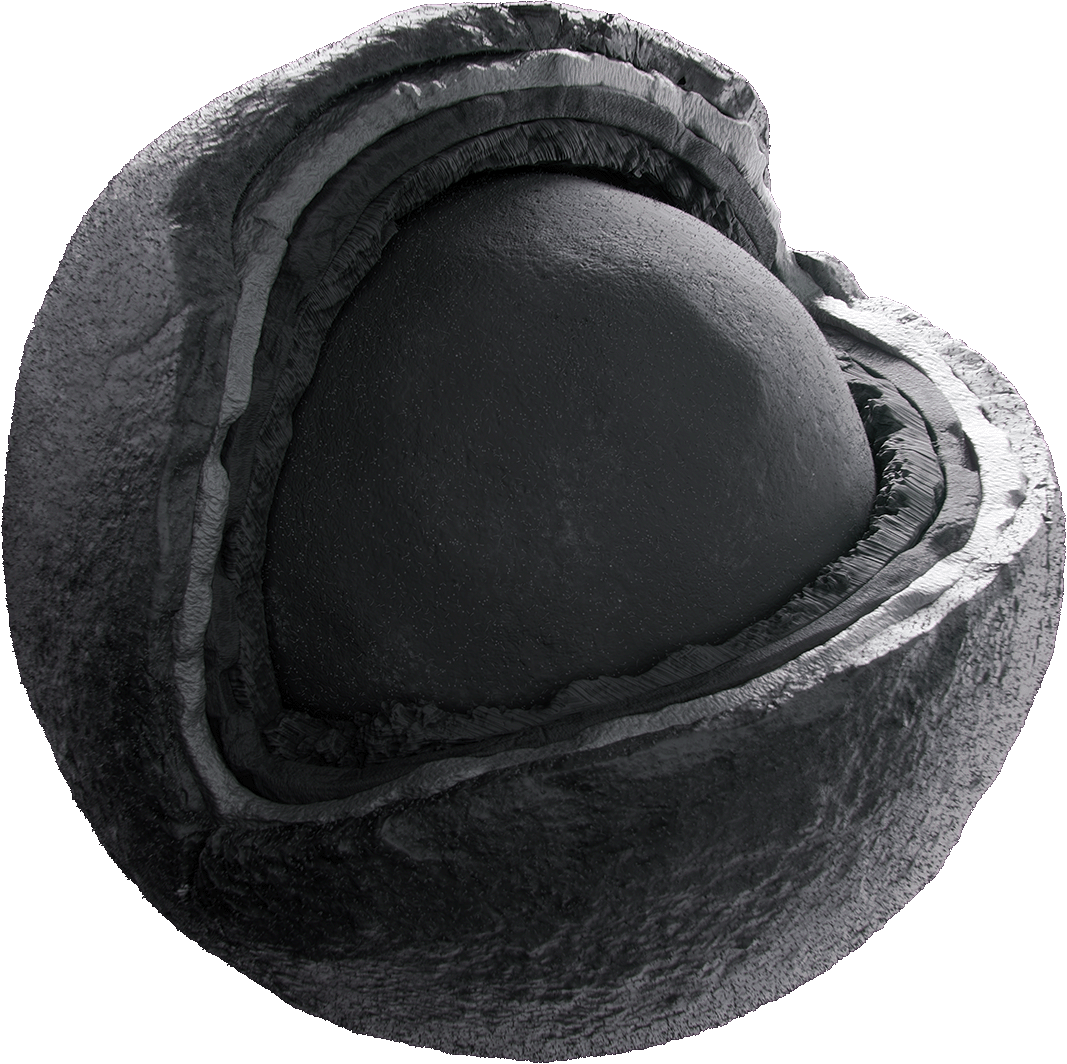
Dense Outer Pyrolytic Carbon
A final outer Pyrolitic carbon (OPyC) layer protects the SiC from further processing steps and provides a compliant layer between the SiC and the matrix that binds the particles into a pellet. The interaction between the outer and inner PyC layers, which shrink during irradiation, provides compressive stress to the Silicon Carbide layer. This means that the SiC starts in compressive stress and transitions to tensile stress as the fission gas pressure increases.
Silicon Carbide
The Silicon Carbide layer acts as the pressure vessel and diffusion barrier for the fission product gases. Microstructurally, smaller equiaxed grains are desired as it lowers diffusion of fission products. These are achieved by using lower temperatures during deposition. SiC is highly radiation tolerant and is effectively impermeable to most of the fission products. Zirconium carbide is a good alternative for higher temperatures.
Dense Inner Pyrolytic Carbon
A denser inner PyC (IPyC) layer is added to protect the fuel kernel from the subsequent processing steps. In particular, the SiC deposition involves hydrochloric acid products which can damage the layers underneath. PyC is also used for its high conductivity at high temperatures, very low thermal expansion, and chemical stability. The PyC is impermeable to most fission products including all the gases and has maximum density to slow down the diffusion of heavy metal fission products like palladium that can the attack SiC.
Porous Pyrolytic Carbon
A porous pyrolytic carbon (PyC) buffer layer (Buffer) acts as a gas chamber for fission product gases and provides sacrificial volume to absorb fission product recoils and any swelling from the fuel kernel. PyC is used for its high conductivity at high temperatures, very low thermal expansion, and chemical stability. Higher porosity is achieved by increasing deposition rate which also increases isotropy.
Fuel Kernel
Our techniques allow for a wide variety of fuel types including Uranium Nitride (UN) and Uranium Oxy Carbide (UCO) as well as thorium based fuel kernels. These advanced fuels allow for more flexible fuel cycles and more capable reactors.
How it's made
The fabrication process is composed of two parts – first, the fabrication of TRISO particles, and second, the fabrication of FCM fuel pellets containing those TRISO particles. The first step is to package Uranium into spherical kernels using the sol-gel process. Uranium oxide is dissolved in nitric acid to make a broth solution of uranyl nitrate. This yellow solution is combined with an organic solution to create a uranium gelation broth used in the sol-gel process.
Uranium Gelation
A liquid uranium broth is cooled to low temperatures and dispersed as droplets into an oil bath. The droplets undergo thermally activated reactions that solidify the droplets into soft gel spheres, a few millimeters in diameter.
Conversion to UCO
The kernels are prepared for a high temperature furnace, to calcine, convert, and sinter mixed uranium trioxide and carbon bearing particles from the sol-gel process to high density uranium oxy-carbide or other types of fuel kernels.
Coating Ceramic Layers
Following recipes tuned over the last several decades, gases are injected into a hot fluidized chamber which can reach temperatures of up to 1700°C. The gases react to deposit chemicals onto the spheres.
Sorting TRISO Particles
One by one, size separation is achieved using roller pins or screens. The rollers are slightly angled so that particles fall through with increasing size. Misshapen or non-spherical particles are sorted using an inclined vibratory plane.
Characterization
TRISO particles and FCM fuel pellets are credited as fission product ‘functional containment’ layers and undergo a rigorous Quality Control Process to ensure that critical parameters are within specification. USNC quality control processes follow closely those developed and used in the U.S. Advanced Gas Reactor Program.
Fuel Forms
USNC's Fully Ceramic Micro-encapsulated fuel form allows for a variety of fuel shapes. TRISO particles can be embedded in a SiC matrix in nearly arbitrary form factors, whether it be annular fuel, cylindrical pellets, or hollow pebbles. It is the fuel of choice for High Temperature Gas-Cooled Reactors (HTGRs), for both prismatic and pebble-bed reactors. Special features on the millimeter size scale can be included, such as alignment features or optimized cooling channels.
Leading Fuel Form for Advanced Reactors
TRISO fuel is one of the leading fuel forms for advanced reactors, small modular reactors (SMRs), and micro reactors. Many other reactor vendors besides USNC are relying on TRISO particle fuel to enhance the safety and reliability of their nuclear reactors. The US DOE is funding the development of multiple TRISO based reactor designs including those from X Energy, BWXT, Westinghouse eVinci, Kairos Power, Boston Atomics, and Radiant Nuclear. US DOD is also using TRISO fuels for the Project Pele transportable micro reactor program. NASA is using TRISO based fuels in both the Lunar Surface Fission Power as well as Nuclear Thermal Propulsion programs.
Fluoride Salt-Cooled Reactor
The Fluoride Salt-Cooled High-Temperature Reactor (FHR) are reactors that use graphite moderator, solid fuels, and molten salt coolant. The most promising versions of the the
Pebble-Bed HTGR
From its first conception during 1940s, High Temperature Gas-Cooled Reactors were originally pursued in Germany, the UK, and the USA. Germany focused on pebble-beds with
Nuclear Gensets
Some microreactor variants are smaller and containerized, potentially allowing for frequent transportability and could have similar characteristics to diesel gensets. These transportable or mobile microreactors
Prismatic HTGR
High temperature gas-cooled reactors (HTGR) come in basically two flavors: prismatic cores and pebble-bed cores. Both use graphite moderator and TRISO fuel particles to make